by Omegan Wright

The world has an $818 billion problem on its hands and, with no solution in sight the problem is projected to grow to two trillion dollars in the next six years. The issue at hand is Alzheimer's disease, a neurodegenerative condition characterized by irreversible, progressive neurological degeneration resulting in memory loss, impaired thinking and reasoning, as well as personality and behavioral changes (Liu et al., 2019). Aging is the primary risk factor in the progression of the disease. For individuals over the age of 65 their chances of developing Alzheimer's doubles every 5 years (Liu et al., 2019). The trend is already being observed globally, as every 20 years, the number of individuals diagnosed with Alzheimer's doubles (Liu et al., 2019). The widespread impact of Alzheimer's disease extends beyond financial costs, creating a significant socio-cultural burden. Families will need to make sacrifices to take care of ailing elderly family members as they can no longer take care of themselves. Alzheimer's disease is an illness that robs people of their autonomy, their past, and their sense of self. Yet, we may have been examining it all wrong. This article is the first in a series focusing on the different theories of Alzheimer's disease pathogenesis. In this installment, we will examine the prevailing theories of the pathogenesis of Alzheimer's disease, their historical and current significance, and the reasons these theories have faced increasing criticism from the broader medical community since 2014.
The Amyloid Cascade Hypothesis: An Issue with Hyperfixation?
The amyloid cascade hypothesis is the second major hypothesis to gain traction in the scientific community regarding Alzheimer’s Disease (AD). In 1991, John Hardy and David Allsop proposed that the rapid deposition in the brain of a protein fragment called Amyloid-β (Aβ) causes the buildup of plaques, which they suspected to be the primary driver of Alzheimer's disease (Liu et al., 2019). These initial findings were based on previous studies regarding AD symptoms observed in individuals with Down's Syndrome who have an extra copy of chromosome 21 leading to overproduction of proteins encoded on that chromosome. Researchers identified a connection between specific genetic mutations in three key genes located on chromosome 21 and the overproduction of amyloid precursor protein (APP), which generates Aβ. As the theory and science evolved, more pathways and mechanisms were included until the amyloid cascade hypothesis outlined a detailed pathway: Aβ deposition leads to the phosphorylation of Tau oligomers, causing plaques and neurofibrillary tangles, as well as resulting in cognitive impairment and degeneration due to neuronal death (Liu et al., 2019). The key feature of this theory is that amyloid accumulation is the initiating event that causes Alzheimer’s disease (Frisoni et al., 2022). This proposed mechanistic pathway is supported by extensive evidence and research, including studies examining the relationship between Aβ presence and AD progression, the temporal associations between Tau and Aβ presenting Aβ as the proceeding factor, and findings that certain forms and quantities of Aβ are toxic (Frisoni et al., 2022). Conversely, alternative evidence gathered over the past few decades has challenged the linearity of this hypothesis..
The first cracks in the hypothesis was the conclusion that accumulation Aβ occurs over a long period of time and isn't indicative of cognitive impairment in some individuals. In autopsies of cognitively normal adults, Aβ dispersion through the liquid environment of the brain matter was fairly common (Yadollahikhales & Rojas, 2023). Although, not at the acute levels observed in AD (Yadollahikhales & Rojas, 2023). It has been determined that approximately 30% of adults with normal cognition have signs of Aβ accumulation or plaque formation implicated as the source of AD pathology (Liu et al., 2019). Additionally, the distribution of Aβ deposition is not consistent with the features of neurodegeneration in Alzheimer’s disease (Roda et al., 2022). The amyloid cascade hypothesis also struggles to explain the 95% of Alzheimer's cases that are sporadic rather than familial AD. Sporadic AD is a multifactorial disease with multiple modulating environmental and genetic variables, with the ε4 allele of apolipoprotein E (APOE) being the most significant genetic risk factor (Liu et al., 2019). Most critically, the clinical trials based on the amyloid cascade hypothesis are, at most, mildly effective, even as they treat the plaque build-up extremely well (Kepp et al., 2023). For instance, Solanezumab, a monoclonal antibody treatment targeted toward the clearance of Monomeric and soluble Aβ, was extremely effective in reducing free plasma Aβ concentrations by more than 90%, but did not lead to significant cognitive improvement (Frisoni et al., 2021; Liu et al., 2019). It also failed stage 3 clinical trials due to significant adverse side effects, and is not currently on the market (Frisoni et al., 2021). Other antibody treatments, such as Aducanumab, Lencecumab, and Donanemab, have been approved recently, but controversy has already arisen. Aducanumab was approved in June 2021 under an accelerated approval protocol based on its ability to remove plaques. It was the first drug approved for AD in 18 years, yet the efficacy of its treatment is questionable (Imbimbo et al., 2023). Since plaque removal only slows the progression of the disease mildly, or rather 22% in this case, and there was a high incidence of side effects, it was recently removed from the market and replaced with Lecanemab (Frisoni et al., 2021; Liu et al., 2019). Lecanemab targeted Aβ protofibrils and showed promising clinical results but did not meet primary endpoints, such as a positive change in dementia related cognitive impairment, in phase two clinical trials; however it did slow cognitive decline with a highest success of all other approved therapies at 47% (Frisoni et al., 2022). Similarly, Donanemab, approved under the FDA’s breakthrough therapy designation, aimed to reduce plaque burden by targeting the Aβ peptides directly (Yadollahikhales & Rojas, 2023). While it reduced plaque levels by 80%, it did not significantly improve outcomes in the four cognitive scales used as the study’s primary endpoint; the best this expensive therapy could do was slow the progression of the disease (Yadollahikhales & Rojas, 2023). The limited success of these therapies has prompted growing skepticism of the amyloid cascade hypothesis. While the hypothesis has undoubtedly contributed to understanding Alzheimer’s disease, its central role as the primary driver of the condition is increasingly questioned.
The Tau Propagation Hypothesis: The Actual Toxin?
The Tau hypothesis posits that the abnormal accumulation of tau protein tangles, known as neurofibrillary tangles (NFTs), disrupts the structural integrity and function of neurons in the central nervous system (CNS), ultimately leading to cell death (Kapoor & Chinnathambi, 2023). The Tau propagation hypothesis was proposed by Frost et al. in 2009 with a study that demonstrated how misfolded Tau could be taken up into cells and propagate in a prion-like manner (Liu et al., 2019; Takeda, 2019). Tau is a very soluble protein commonly found in both the peripheral and central nervous systems, and crucial in stabilizing the microtubules and facilitating cellular transport (Kapoor & Chinnathambi, 2023). However, Tau can also spread in a prion-like manner. Neurons already hosting dysfunctional tau can spread then to healthy neurons releasing tau "seeds", oligomers of misfolded Tau, that can be taken up by neighboring cells. When the dysfunctional Tau comes into contact with functional Tau in the typical neuron, it confers this dysfunction (Pascoal et al., 2021). Dysregulated Tau is often caused by genetic mutations or post-translational modifications such as phosphorylation after assembly, which alters the protein's structure, making it more prone to aggregation and NFT formation. (Roda et al., 2022). Hyperphosphorylated Tau aggregates into paired helical filaments that clump together into NFTs, also called tangles. NFTs are problematic as they cause disruptions in critical components of normal neuronal function, including axonal transport, synaptic transmission, and neuronal signaling. The breakdown of these processes can kill the neuron, contributing significantly to the cognitive decline observed in individuals with AD (Kapoor & Chinnathambi, 2023). What makes the Tau hypothesis particularly compelling is its alignment with the progression of Alzheimer’s disease unlike the AB deposition hypothesis. The spread of Tau tangles closely correlates with disease stages, as reflected in Braak staging (I-VI), a model consistently validated across studies (Kapoor & Chinnathambi, 2023; Pascoal et al., 2021). This temporal and spatial alignment strengthens the argument that Tau is a primary driver of neurodegeneration in Alzheimer’s disease.
Despite its appeal, the Tau propagation hypothesis is not without limitations. There are some gaps to be explored and difficulty in executing clinical treatments based on these observations. The mechanism doesn’t exactly mediate the connection between tau and Aβ plaques, In the mesial temporal regions of the brain—where Tau dysregulation often begins—low levels of Aβ are present and are believed by some to initiate the dysfunction (Pascoal et al., 2021). Additionally, the microglial cells of the CNS have been implicated in the initiation of tau propagation. This suggests that while Tau plays a critical role in disease progression, it may not be the initiating factor (Pascoal et al., 2021). Also, tau-target therapies are hard to develop because they exist in six isoforms in the human brain, and their levels become dysregulated during Alzheimer’s progression, lowering targeting efficacy (Roda et al., 2022,). Furthermore, the precise mechanisms behind Tau hyperphosphorylation and subsequent aggregation into NFTs remain mostly unknown, so highly specific treatments cannot be developed at this time (Roda et al., 2022). Another concern is that by the time Alzheimer’s disease is diagnosed, the damage may be too advanced for monotherapies targeting Tau to reverse its progression, and treatment of a singular contributing factor might not be enough to salvage the situation. Still, the Tau propagation hypothesis provides a compelling alternative to the amyloid cascade hypothesis, especially given its closer alignment with Alzheimer’s disease progression. However, the theory faces significant scientific and therapeutic challenges that must be addressed.

Figure 1. Depicts the location of Tau (red dots) relative to the anatomical damage associated with Alzhimer’s disease progression. The darker the region of the brain the more damaged the area. The darker the dot the higher concentration of deposits there are in the area.
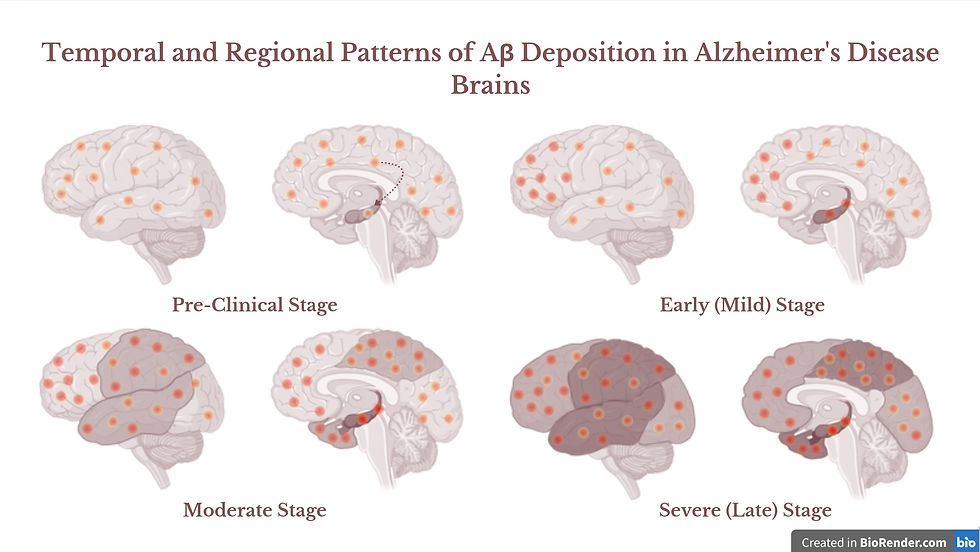
Figure 2. Depicts the location of Aβ (red dots) relative to the anatomical damage associated with Alzheimer’s disease progression. The darker the region of the brain the more damaged the area. The darker the dot the higher concentration of deposits there are in the area.
The Neuroinflammation Hypothesis: A Slippery Slope
Inflammation is an essential physiological process, enabling the body to combat injury and infection; the surrounding tissue fills with fluid medium, like cerebrospinal fluid, to facilitate the transport of healing metabolites and immune cells that can fight off potential infections. However, prolonged inflammation can be detrimental and cause the death of the cells in that area (Wang et al., 2023). This effect is especially pronounced in the brain, where each neuron plays a vital role in overall function. Microglia and astrocytes are responsible for inflammatory responses in the brain. More specifically, microglial cells are integral to brain health at all stages, and, in the case of non-injury, they primarily scan the surrounding area waiting to be activated (Wang et al., 2023). However, once activated, microglia can polarize into two distinct states: the mobile, pro-inflammatory M1 state and the anti-inflammatory M2 state (Wang et al., 2023). In AD, the M1 form predominates, spreading inflammation-promoting metabolites like cytokines, chemokines, etc. (Wang et al., 2023). When it comes to the relationship between microglia and the progression of AD, the temporal lines between hallmarks of the disease get complex. Activated microglia not only promote the aggregation of Aβ and Tau but are also influenced by these proteins, creating a vicious cycle. Evidence suggests that microglial activation and the resulting neuroinflammatory response may precede Aβ and Tau pathologies in some cases (Calsolaro & Edison, 2016). For example, mutations in the TREM2 gene, which encodes a receptor on microglia that regulates inflammation, are more prevalent in AD patients. These mutations impair TREM2’s ability to suppress cytokine production and mediate inflammatory responses, allowing unchecked inflammation even in the absence of significant Aβ or Tau changes (Calsolaro & Edison, 2016). The mutation triples the risk of development of late-onset AD (Calsolaro & Edison, 2016). Dark or dystrophic microglia are also a newly discovered phenotype of glial, characterized by aberrations in the chromatin, tightly wound DNA (Wang et al., 2023). Dark microglia have been observed to precede the spread of Tau pathology, indicating an interesting and novel temporal landmark (Wang et al., 2023). Epidemiological data supports clinical systemic inflammation as a risk factor which is strongly associated with the development of AD as well as correlated conditions like obesity, diabetes, cardiovascular disease, and metabolic syndrome (Calsolaro & Edison, 2016). Interestingly, prolonged use of non-steroidal anti-inflammatory drugs (NSAIDs) appears to provide some protection against AD, although clinical trials have yielded mixed results (Calsolaro & Edison, 2016). Though, in the literature review, the authors still attempted to characterize Aβ as the precursor to most mechanisms, which could be due to an adherence to upheld dogma or a lack of concrete clinical evidence allowing them to shift their hypothesis effectively.
Potential treatments in this vector may be more effective than the previously mentioned Tau targeted and Aβ as it might mitigate damage being proliferated. Prospective medical interventions could include suppressing microglial priming in the pre-clinical phase of the disease, modulating microglia polarization to a more anti-inflammatory phenotype, and limiting the pro-inflammatory response of the microglia (Wang et al., 2023). These options include novel or well-researched targets and treatments, like statins, antioxidant supplementation (folic acid, omega-3 fatty acids), NF-kB, etc. (Wang et al., 2023). It bypasses the targeting issue seen in Tau and the miscorrelation between cognitive decline and Aβ plaques. However, these approaches are not without risks. Using agents that would inhibit the natural immune progress of the brain, such as microglia modulation or limiting the proinflammatory response, could leave individuals vulnerable to infections, and infections in the brain are particularly devastating for the same reasons cell death in the brain is devastating.
Trying to See the Bigger Picture
The Amyloid cascade, tau progression, and neuroinflammation hypotheses each highlight vital aspects of Alzheimer’s disease (AD) progression but fall short of crafting a definitive antithesis to the development of AD. To truly understand AD, we must widen the scope of inquiry, integrate diverse theories, examine interwoven mechanisms, and challenge entrenched perspectives. A unified understanding of all the factors at play in this disease is going to prove necessary for the comprehension and development of an effective treatment and, eventually, a cure. In the next article in this series, we will explore new hypotheses as well as up-and-coming ideas, aiming to build a more comprehensive understanding of this increasingly prevalent disease. Stay tuned!
References
Calsolaro, V., & Edison, P. (2016). Neuroinflammation in Alzheimer's disease: Current evidence and future directions. ALZHEIMERS & DEMENTIA, 12, 719-732. https://doi.org/10.1016/j.jalz.2016.02.010
Frisoni, G., Altomare, D., Thal, D., Ribaldi, F., van der Kant, R., Ossenkoppele, R.,…Dubois, B. (2022). The probabilistic model of Alzheimer disease: the amyloid hypothesis revised. NATURE REVIEWS NEUROSCIENCE, 23, 53-66. https://doi.org/10.1038/s41583-021-00533-w
Imbimbo, B., Ippati, S., Watling, M., & Imbimbo, C. (2023). Role of monomeric amyloid-β in cognitive performance in Alzheimer's disease: Insights from clinical trials with secretase inhibitors and monoclonal antibodies. PHARMACOLOGICAL RESEARCH, 187, Article 106631. https://doi.org/10.1016/j.phrs.2022.106631
Kapoor, M., & Chinnathambi, S. (2023). TGF-β1 signalling in Alzheimer's pathology and cytoskeletal reorganization: a specialized Tau perspective. JOURNAL OF NEUROINFLAMMATION, 20, Article 72. https://doi.org/10.1186/s12974-023-02751-8
Kepp, K., Robakis, N., Hoilund-Carlsen, P., Sensi, S., & Vissel, B. (2023). The amyloid cascade hypothesis: an updated critical review. BRAIN, 146, 3969-3990. https://doi.org/10.1093/brain/awad159
Liu, P., Xie, Y., Meng, X., & Kang, J. (2019). History and progress of hypotheses and clinical trials
for Alzheimer's disease. SIGNAL TRANSDUCTION AND TARGETED THERAPY, 4, Article 29. https://doi.org/10.1038/s41392-019-0063-8
Pascoal, T., Benedet, A., Ashton, N., Kang, M., Therriault, J., Chamoun, M.,…Rosa-Neto, P. (2021). Microglial activation and tau propagate jointly across Braak stages. NATURE MEDICINE, 27, 1592-+. https://doi.org/10.1038/s41591-021-01456-w
Roda, A., Serra-Mir, G., Montoliu-Gaya, L., Tiessler, L., & Villegas, S. (2022). Amyloid-beta peptide and tau protein crosstalk in Alzheimer's disease. NEURAL REGENERATION RESEARCH, 17, 1666-1674. https://doi.org/10.4103/1673-5374.332127
Takeda, S. (2019). Tau Propagation as a Diagnostic and Therapeutic Target for Dementia: Potentials and Unanswered Questions. Front Neurosci, 13, 1274. https://doi.org/10.3389/fnins.2019.01274
Wang, C., Zong, S., Cui, X., Wang, X., Wu, S., Wang, L.,…Lu, Z. (2023). The effects of microglia-associated neuroinflammation on Alzheimer's disease. FRONTIERS IN IMMUNOLOGY, 14, Article 1117172. https://doi.org/10.3389/fimmu.2023.1117172
Yadollahikhales, G., & Rojas, J. (2023). Anti-Amyloid Immunotherapies for Alzheimer's Disease: A 2023 Clinical Update. NEUROTHERAPEUTICS, 20, 914-931. https://doi.org/10.1007/s13311-023-01405-0
Comments